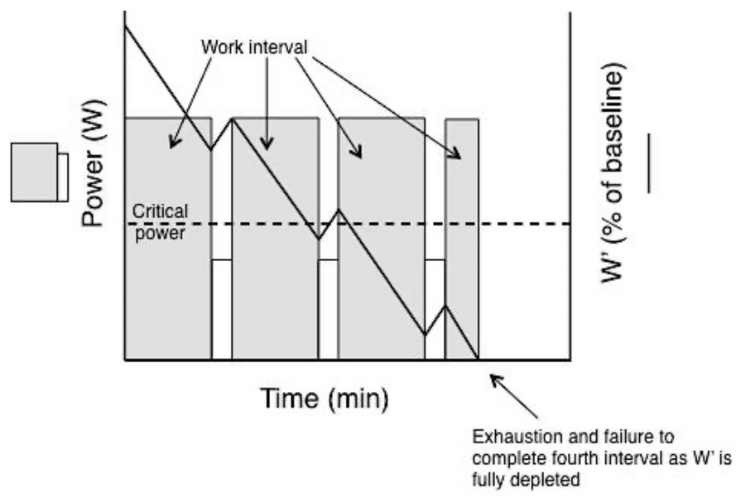
Endurance training 2: High intensity interval training
Building on the basic principles of endurance training adaptation we discussed last week, in this blog we are going to cover the basics of something I am sure is familiar to all readers – high-intensity interval training. One way or another, anyone training for endurance sport should include high- intensity interval training in and throughout their structured programme.
Why? Well, high-intensity interval training is one of the best-supported practices in endurance sport. This type of training has been studied by countless research groups around the world, with consistent positive effects reported for physiological adaptation and, importantly, endurance performance.
In our last blog, we discussed how training stresses the body and disturbs its homeostasis, which provides the signal for the beneficial adaptations that make us better at endurance sport. High-intensity interval training, by definition, is designed to induce large homeostatic disturbances in a short period of time. Indeed, an excellent study published last year showed that the signal generated for adaptation is proportional to the homeostatic disturbance, with the largest signals generated when the homeostatic disturbance is greatest (8). Moreover, a recent synthesis of all available data suggested certain physiological adaptations are intensity-dependent and therefore much more pronounced with the higher training intensities seen during interval training (9). So, what exactly is high-intensity interval training, and how do we apply it into our Elevate Coaching training plans?
What is high-intensity interval training?
High-intensity interval training refers to sessions including multiple defined bouts of exercise above the anaerobic threshold, with programmed recovery in between. The anaerobic threshold, another really important concept for endurance training, refers to the highest exercise intensity at which a steady- state physiology can occur. This is often called the lactate threshold or critical power (itself a concept we will discuss later) and is estimated with some, but not great, accuracy by the 20-min FTP (functional threshold power) test (2, 10, 14, 15). Importantly, then, these high-intensity intervals above the anaerobic threshold cannot be sustained for long periods, as they are characterised by a continually rising level of oxygen consumption and blood lactate concentration, and continually falling muscle and blood pH (indicative of greater and greater acidity) and anaerobic fuel stores such as phosphocreatine. Intervening recovery periods then allow for full or partial recovery of these physiological variables prior to the start of the next interval. These training sessions are an efficient means of disturbing homeostasis to generate endurance adaptations (3).
Example high-intensity interval training sessions for a cyclist with an anaerobic threshold of 300 W may therefore be as follows:
-
8x3min at 330W, with 2min easy spinning at~100W between-reps
-
8x1min at 360W, with1min easy spinning at~100W between-reps
-
4x30sec all-out, with 4min easy spinning at~100W between-reps
-
10 x 10 sec at 400 W, with 50 sec easy spinning at ~100 W between- reps
Types of high-intensity interval training sessions
The sessions described above fall into distinct categories of high-intensity interval training: long, short, sprint, and repeated sprint, respectively. These separate types of interval training evoke different physiological stresses and are therefore programmed according to the goals of the athlete. For instance, long intervals such as the first session listed above evoke substantial strains on our aerobic metabolism, due to the prolonged period spent at high-intensity in each repetition. In contrast, the sprint interval training session listed third is a strong stimulus for our neuromuscular system, as we have to activate a large amount of muscle mass to produce the very high ‘all-out’ power output.
Practically speaking, long intervals involve repetitions of greater than 1 min, with shorter recovery durations, whereas short intervals last up to a minute with similar recovery durations. Sprint interval training sessions involve a small number of short ‘all-out’ sprint efforts with recovery durations much longer than the work periods, and repeated sprint sessions are generally not quite all-out, but instead involve short bursts of power with recovery periods of up to a minute in duration.
Application of high-intensity interval training
So, other than ensuring work intervals take place above the anaerobic threshold, and selecting the appropriate type of interval training session, how do we go about programming and progressing interval training sessions? To do this well, it’s important to consider the key factors that can be manipulated when building an interval training session: interval intensity and duration, and recovery intensity and duration, as altering these variables produces divergent physiological responses and allows us to achieve different goals (3).
To understand how this works, it is useful to consider high-intensity interval training under the ‘critical power’ model of endurance (12). This model has
been constructed based on a large number of laboratory studies measuring physiological responses and tolerable durations at different workloads (13, 19, 20), and has identified two key variables: critical power and W’ (12, 21). Understanding these variables gives us a strong conceptual basis for programming and progressing our high-intensity interval training sessions.
Critical power refers to the highest exercise intensity at which a ‘steady-state’ in oxygen consumption and blood lactate concentration can be attained, thus is conceptually very similar to the anaerobic threshold I described above (11). At or below critical power, these variables will rise and then level off before exhaustion, which generally occurs after ~30-60 min when exercising at critical power (1). When exercising above critical power, oxygen consumption, blood lactate concentration, and heart rate progressively and continually rise, and muscle phosphocreatine stores and pH progressively decrease, until exhaustion occurs (1, 13, 17, 19). Fatigue during exercise performed above critical power seems to occur after a set absolute amount of ‘work above critical power’, measured in kilojoules, has been performed – the so-called W’, which of course will vary between-athletes. This means that fatigue in an individual athlete performing exercise above his or her own critical power occurs after their W’ has been expended. Hopefully you can see these concepts in the Figure below.
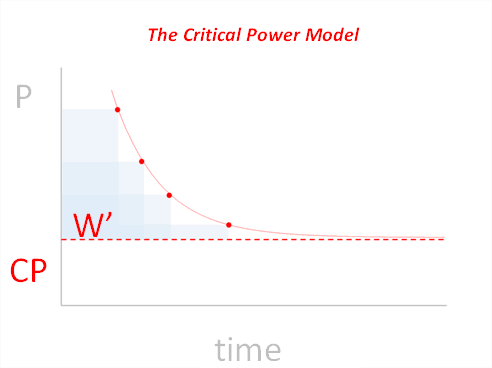
Fig. 1. The critical power concept. Critical power is the maximum intensity at which steady-state physiology can be achieved, and exhaustion is reached during exercise performed above critical power after a set amount of ‘work above critical power’ (W’) is expended.
Work intervals above critical power during high-intensity interval training, then, partially expend W’, which is then partially or fully recovered during the subsequent recovery period (16), with the rate at which this W’ recovery occurs dependent on how easy and how long the recovery period between- intervals is (4, 5, 7). These factors therefore need to be considered at an individual level to ensure that the desired response is seen during a given interval training session. For example, consider the following example 4 x 3- min high-intensity interval training session in the same athlete.
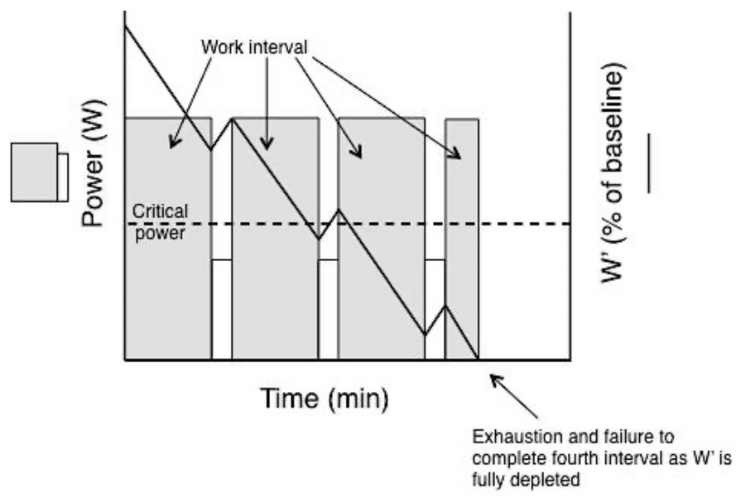
As you can see, this athlete failed to complete the fourth 3-min repetition as their W’ was fully depleted just 90 seconds into the last interval. In this case, it appears that insufficient W’ recovery occurred during the recovery period due to the recovery period intensity being too high (i.e. too close to critical power). By reducing the recovery period intensity, greater W’ recovery is achieved, allowing the fourth interval to be completed prior to full W’ depletion and exhaustion. See below.
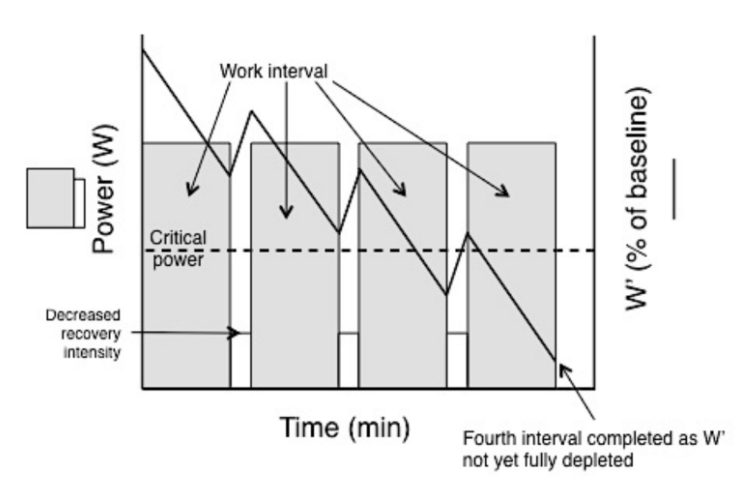
Therefore, by successful manipulation of the key high-intensity interval training parameters, the 4 x 3 min session could be completed at the desired power output. These are just some of the considerations we have to make as endurance athletes and coaches when designing training sessions to achieve our goals.
As the athlete gets fitter, we are likely to want to progress these sessions in line with the fundamental training concept of progressive overload. To do so, and depending on our goal, we could increase the power output during the work intervals, increase the duration of the intervals, or increase the number of repetitions performed.
Summary: Will high-intensity interval training make me faster?
So, hopefully I have outlined that high-intensity interval training is an efficient means of disturbing homeostasis to trigger positive endurance adaptations, and described some of the fundamental considerations we have to make when programming high-intensity interval training sessions, along with the conceptual basis behind these considerations. But, does the science show that doing this type of very demanding training will actually make me faster?
The short answer to this question is a resounding “yes”. Countless studies have been published in this regard, with the balance of evidence heavily in favour of an ergogenic effect of performing some high-intensity interval training (3, 6, 18). That is why we consider high-intensity interval training, in at least one of its types, to be one of the cornerstones of a well-structured endurance training programme aimed at maximising the performance and health benefits of training.
However, and crucially, that is not to say that all of our training should be at high intensities or involve high-intensity intervals. How frequently we should perform high-intensity interval training, and the specific value of low-intensity training, is the subject of the next blog. Stay tuned!
References
-
Black MI, Jones AM, Blackwell JR, Bailey SJ, Wylie LJ, McDonagh STJ, Thompson C, Kelly J, Sumners P, Mileva KN, Bowtell JL, Vanhatalo A. Muscle metabolic and neuromuscular determinants of fatigue during cycling in different exercise intensity domains. J Appl Physiol 122: 446–459, 2017.
-
Borszcz FK, Tramontin AF, Costa VP. Is the functional threshold power interchangeable with the maximal lactate steady state in trained cyclists? Int J Sports Physiol Perform 14: 1029–1035, 2019.
-
Buchheit M, Laursen PB. High-intensity interval training, solutions to the programming puzzle: Part I: Cardiopulmonary emphasis. Sport Med 43: 313–338, 2013.
-
Chidnok W, Dimenna FJ, Bailey SJ, Vanhatalo A, Morton RH, Wilkerson DP, Jones AM. Exercise tolerance in intermittent cycling: Application of the critical power concept. Med Sci Sports Exerc 44: 966– 976, 2012.
-
Chidnok W, DiMenna FJ, Fulford J, Bailey SJ, Skiba PF, Vanhatalo A, Jones AM. Muscle metabolic responses during high-intensity intermittent exercise measured by 31P-MRS: Relationship to the critical power concept. Am J Physiol - Regul Integr Comp Physiol 305: R1085– R1092, 2013.
-
Esfarjani F, Laursen PB. Manipulating high-intensity interval training: Effects on VO2 max, the lactate threshold and 3000 m running performance in moderately trained males. J Sci Med Sport 10: 27–35, 2007.
-
Ferguson C, Rossiter HB, Whipp BJ, Cathcart AJ, Murgatroyd SR, Ward SA. Effect of recovery duration from prior exhaustive exercise on the parameters of the power-duration relationship. J Appl Physiol 108: 866–874, 2010.
-
Fiorenza M, Gunnarsson TP, Hostrup M, Iaia FM, Schena F, Pilegaard H, Bangsbo J. Metabolic stress-dependent regulation of the mitochondrial biogenic molecular response to high-intensity exercise in human skeletal muscle. J Physiol 596: 2823–2840, 2018.
-
Granata C, Jamnick NA, Bishop DJ. Training-induced changes in mitochondrial content and respiratory function in human skeletal muscle. Sport Med 48: 1809–1828, 2018.
-
Inglis EC, Iannetta D, Pass L, Murias JM. Maximal lactate steady state versus the 20-minute functional threshold power test in well- trained individuals: “ Watts ” the big deal? Int J Sports Physiol Perform : 1–7, 2019.
-
Jones AM, Burnley M, Black MI, Poole DC, Vanhatalo A. The maximal metabolic steady state: redefining the ‘gold standard.’ Physiol Rep 7: 1–16, 2019.
-
Jones AM, Vanhatalo A. The ‘critical power’ concept: Applications to
-
sports performance with a focus on intermittent high-intensity exercise.
-
Sport Med 47: 65–78, 2017.
-
Jones AM, Wilkerson DP, DiMenna F, Fulford J, Poole DC. Muscle
-
metabolic responses to exercise above and below the “critical power” assessed using 31P-MRS. Am J Physiol - Regul Integr Comp Physiol 294: R585–R593, 2008.
-
Lillo-Bevia JR, Courel-Ibanez J, Cerezuela-Espejo V, Moran- Navarro R, Martinez-Cava A, Pallares JG. Is the functional threshold power a valid metric to estimate the maximal lactate steady state in cyclists? J. Strength Cond. Res. (2019). doi: doi: 10.1519/JSC.0000000000003403.
-
Morgan PT, Black MI, Bailey SJ, Jones AM, Vanhatalo A. Road cycle TT performance: Relationship to the power-duration model and association with FTP. J Sports Sci 37: 902–910, 2019.
-
Morton RH, Billat VL. The critical power model for intermittent exercise. Eur J Appl Physiol 91: 303–307, 2004.
-
Poole DC, Ward SA, Gardner GW, Whipp BJ. Metabolic and respiratory profile of the upper limit for prolonged exercise in man. Ergonomics 31: 1265–1279, 1988.
-
Seiler S, Jøranson K, Olesen B V., Hetelid KJ. Adaptations to aerobic interval training: interactive effects of exercise intensity and total work duration. Scand J Med Sci Sport 23: 74–83, 2013.
-
Vanhatalo A, Black MI, DiMenna FJ, Blackwell JR, Schmidt JF, Thompson C, Wylie LJ, Mohr M, Bangsbo J, Krustrup P, Jones AM. The mechanistic bases of the power–time relationship: muscle metabolic responses and relationships to muscle fibre type. J Physiol 594: 4407–4423, 2016.
-
Vanhatalo A, Doust JH, Burnley M. Determination of critical power using a 3-min all-out cycling test. Med Sci Sports Exerc 39: 548–555, 2007.
-
Vanhatalo A, Jones AM, Burnley M. Application of critical power in sport. Int J Sports Physiol Perform 6: 128–136, 2011.